Welcome
The atomic nucleus is a complex mesoscopic many-body system where collective and single-particle degrees of freedom coexist. We know nowadays that most heavier nuclei are produced in either charged-particle, neutron-capture or photo-dissociation reactions (and successive radioactive decays) taking place in often explosive stellar scenarios. While many new neutron-rich nuclei will become experimentally accessible with upcoming rare isotope beam facilities and enable the study of lighter to medium-mass rapid neutron-capture process (r process) nuclei near the neutron dripline, many species and their properties will only be accessible by means of theoretical calculations. The latter will then provide the nuclear physics inputs needed for nucleosynthesis network calculations to understand the abundance pattern of isotopes and to identify sites for their possible synthesis. This also means that those nuclear structure models need to be tested in regions where nuclear properties are accessible and can discriminate between competing model descriptions. These tests can and should involve changing ground-state properties as well as characteristics of excited states of both collective and single-particle nature.
The research group of Dr. Spieker performs experiments to study different nuclear-structure phenomena, which could also influence reactions taking place in explosive stellar scenarios. The experiments are performed at the John D. Fox Laboratory at Florida State University. At the heart of the program stands the FSU Enge Split-Pole Spectrograph. A magnetic spectrometer which allows for detailed studies of excited states of the atomic nucleus due to its excellent energy resolution. γ-decay properties of excited states can be selectively studied in particle-γ coincidence experiments. For these experiments, the group has built the prototype of a highly-efficient CeBr3 scintillator array, called CeBrA. The group has also very successfully performed nuclear-structure experiments with GRETINA, the S800 spectrograph and the NSCL/Ursinus Liquid Hydrogen Target at the National Superconducting Cyclotron Laboratory (NSCL) of Michigan State University and was part of first experiments performed with GRETINA at the Facility for Rare Isotope Beams (FRIB) at Michigan State University. Plans to continue the group's program with rare-isotope beams at FRIB are in place.
The group's research is supported by the Department of Physics, Florida State University, the National Science Foundation (NSF) under grant Nos. PHY-2012522 (WoU-MMA: Studies of Nuclear Structure and Nuclear Astrophysics), PHY-2412808 (Studies of Nuclear Structure and Nuclear Astrophysics), and PHY-2405485 (MRI: Equipment: A New CeBr3 Gamma-Ray Detection Array (CeBrA) for Particle-Gamma Coincidence Experiments at the FSU Super-Enge Split-Pole Spectrograph), and by the U.S. Department of Energy, National Nuclear Security Administration (NNSA) under Grant No. DE-NA0004150 [Center for Excellence in Nuclear Training And University-based Research (CENTAUR)] as part of the Stewardship Science Academic Alliances Centers of Excellence Program.
email: mspieker at fsu.edu phone: (850)644-2066 office: Department of Physics, Keen Bldg., Room 217
Current Members of the Research Group
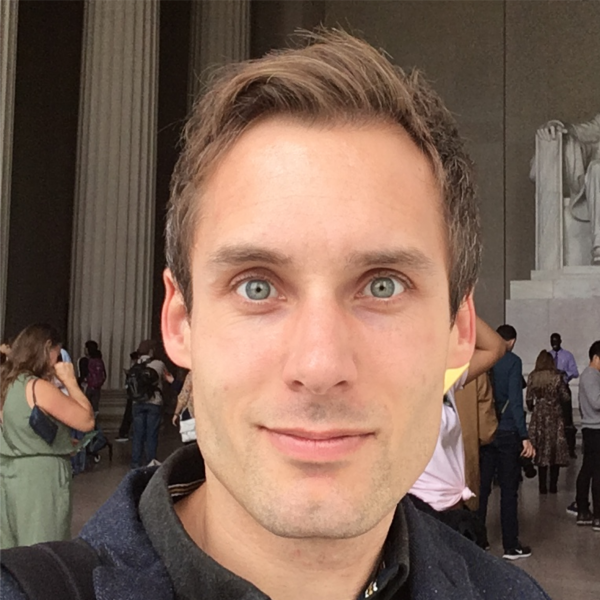
Dr. Mark Spieker
Assistant Professor
Joined FSU in Oct. 2019
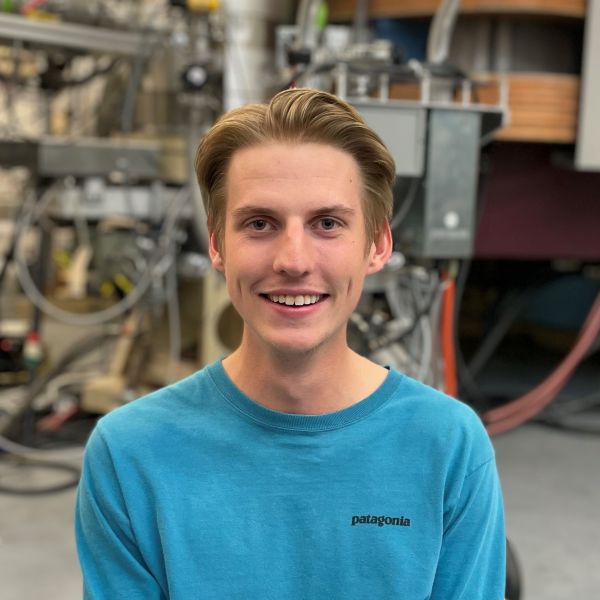
Alex Conley
Graduate Student
Joined group in Feb. 2021
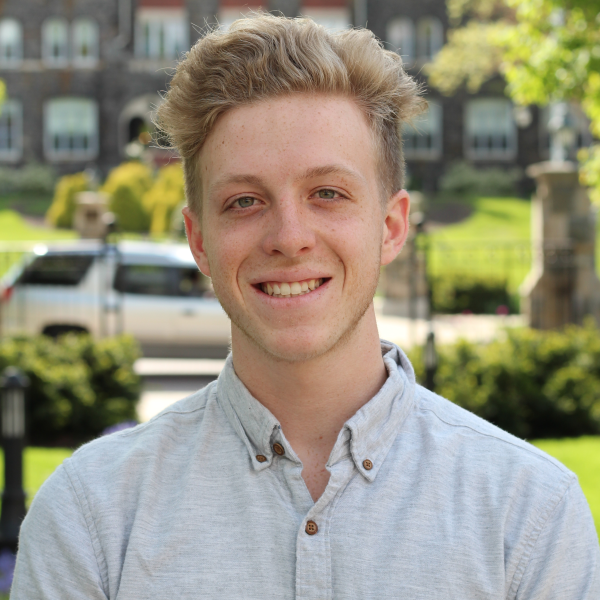
Bryan Kelly
Graduate Student
Joined group in Feb. 2021
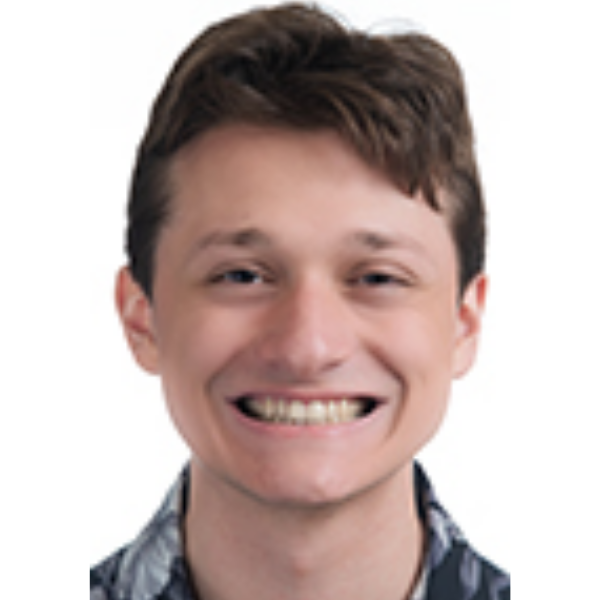
Tyler Stuck
Graduate Student
Joined group in May 2025
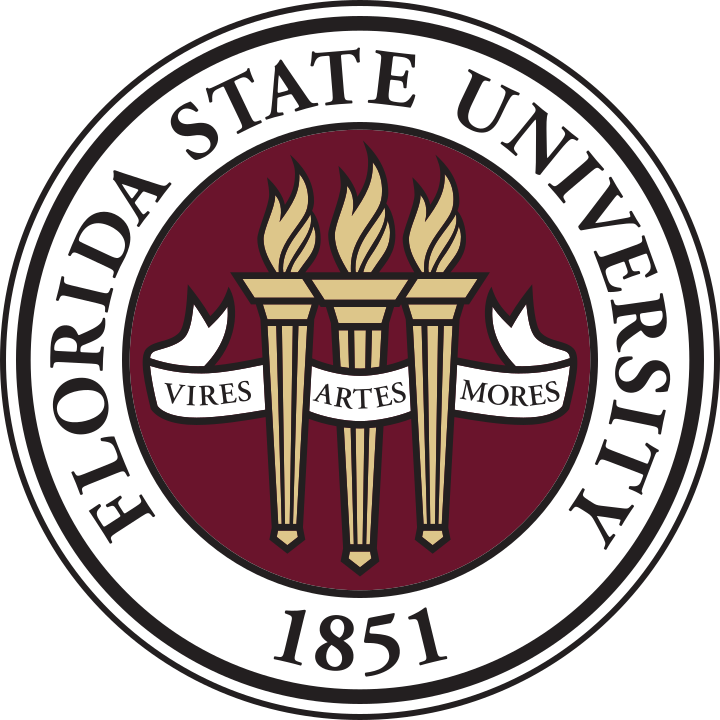
Timothy Kirk
Undergraduate Student
Chemical Engineering
Joined group in Fall 2024
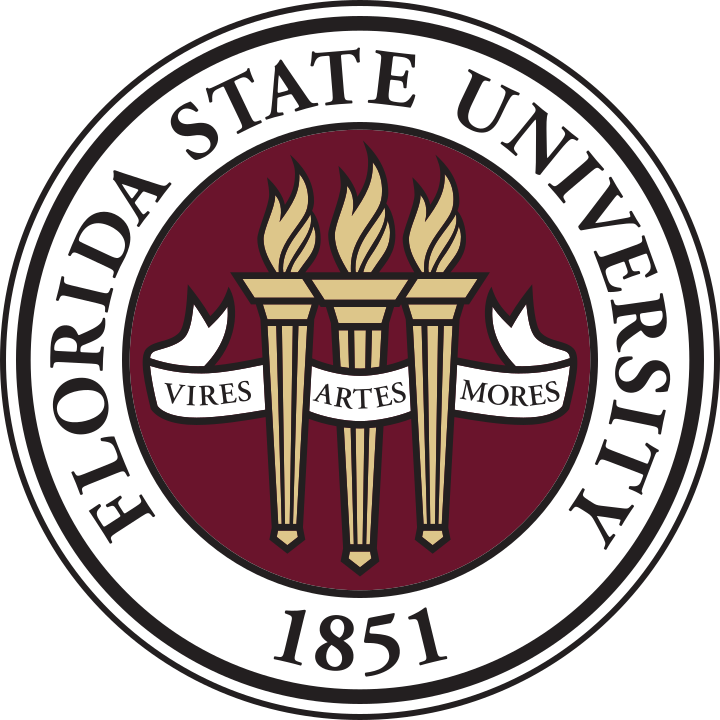
John River Sheridan
Undergraduate Student
Physics
Joined group in Spring 2025
Past Members of the Research Group
Scott Baker, undergraduate student (physics), honors in the major, bachelor's degree [2022-2024]
Emma Crowe, undergraduate student (physics, now electrical engineering) [2024]
Dennis Houlihan, graduate student (physics), master's degree [2022-2024]
Ramiro Renom, undergraduate student (applied mathematics), bachelor's degree [2021-2022]

Research Projects in Our Group
Currently, no open research-assistant positions with my group are available. Check with the other professors working at the John D. Fox Laboratory if you are interested in low-energy nuclear physics and nuclear astrophysics.
Fission-fragment correlations and fission probability distributions measured in light-ion induced fission of actinide nuclei
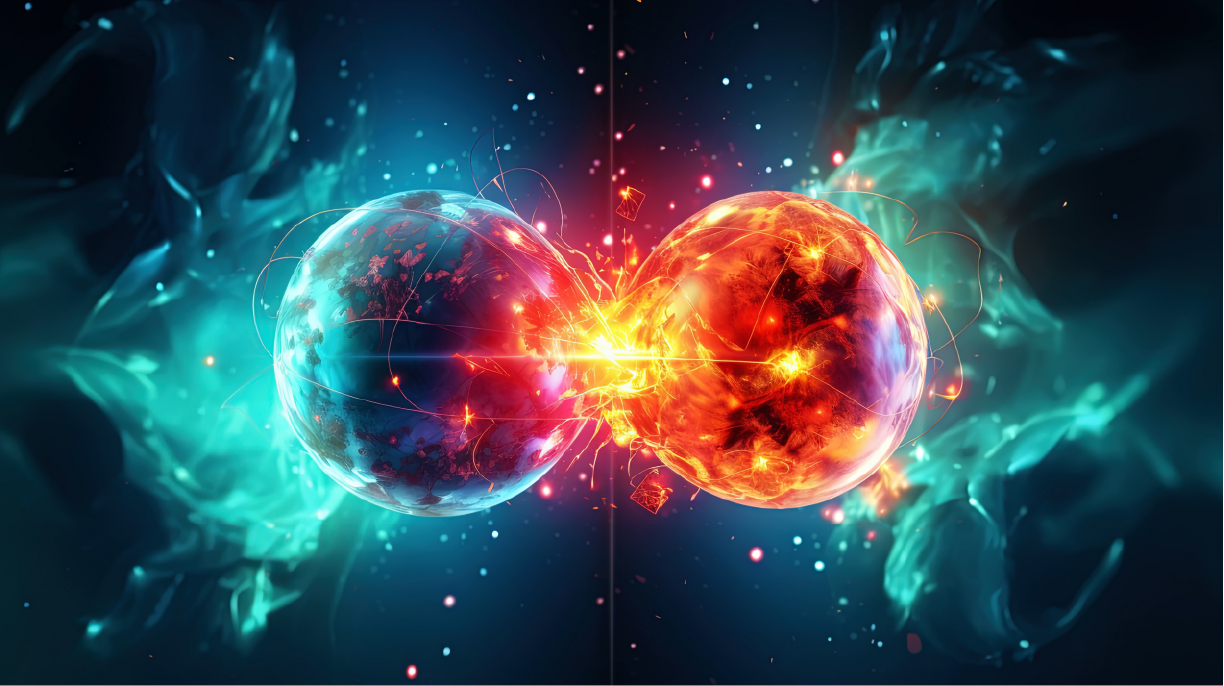
Artistic depiction of nuclear fission (picture credit: Adobe Stock). Nuclear fission is one of the oldest problems in nuclear science. Because of advances in state-of-the-art theory, new questions have recently been asked about the fission dynamics and correlations between the fission fragments. State-of-the-art experiments coupling different detectors can possibly address these open questions.
Detector-development project
This NNSA-supported program to indirectly study the neutron-induced fission of 240U will use the triton beam at the FSU laboratory, which is currently being developed, in conjunction with the SE-SPS spectrograph. A compact solid-state detector array, serving as a fission detector, will be built within the scope of the project. A design similar to the SCARY array, which was used at the Wright Nuclear Structure Laboratory, or to the SORCERER solar-cell array, which is being used at the IFIN-HH, is envisioned.
The planned 238U(t,pf) experiment will establish a fission probability distribution as a function of excitation energy. Measuring angular distributions with the SE-SPS will provide access to a possible spin dependence of the fission probabilities. Particle-fission-fragment angular correlations can also be measured thanks to the segmentation of the planned fission detector. Problems of past (t,pf) experiments with Oxygen and Carbon contaminants can be effectively mitigated by performing measurements with the SE-SPS at different angles (should there be any problems), which will shift the contaminants’ position in the focal plane, and, thus, allow to determine the fission probabilities at various angles for consistency checks.
A new focal-plane detector for the Super-Enge Split-Pole Spectrograph (SE-SPS)
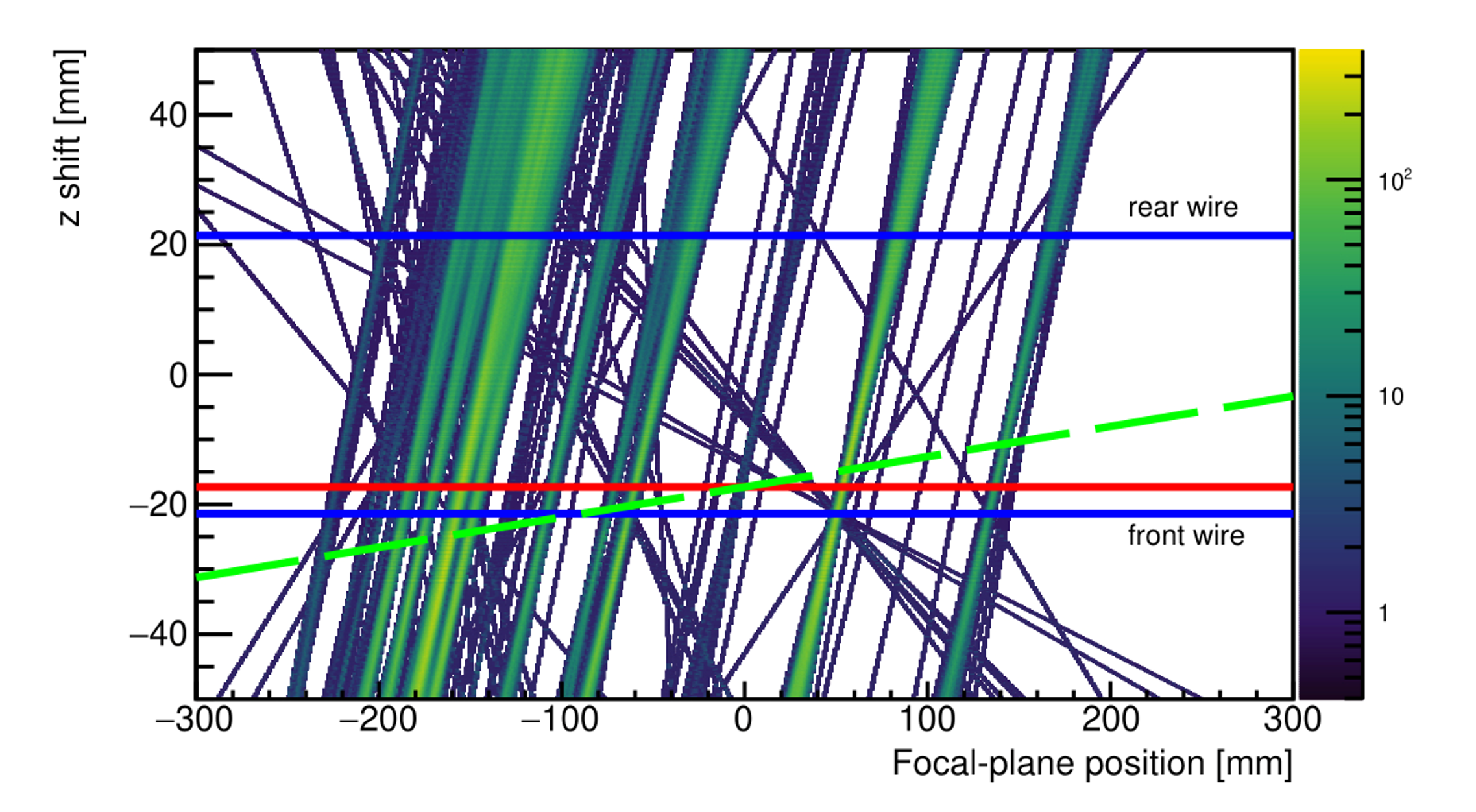
Tracking particles through the focal plane of the SE-SPS with a position sensitive focal plane detector. Currently, two position sensitive anode wires allow to reconstruct the track achieving a position resolution of about 2 mm.
Detector-development project
The current focal-plane detector consists of a position-sensitive proportional counter with two anode wires, separated by about 4.3 cm, to measure position, angle, and energy loss, and a large plastic scintillator to determine the rest energy of the residual particles passing through the detector. Under favorable conditions, the detector can be operated at rates as high as two kilocounts/s (kcps). Both anode wires are inductively coupled to pick-up pads that are read out at the left and right ends of delay lines. The measured time differences allow to track particles through the focal plane of the SE-SPS. As the resolution depends on the solid angle, target thickness and beam-spot size, it may vary from experiment to experiment. In standard operation and with a global kinematic correction, i.e., assuming a vertical shift of the real focal plane with respect to the two position-sensitive sections of the detector, a full width at half maximum of 30-70 keV has been routinely achieved (see red line in figure). This corresponds to a position resolution of about two millimeters. This resolution can be improved further with position-dependent offline corrections, e.g., assuming a linear dependence of the z shift on the focal-plane position (green, dashed line in figure).
To overcome current limitations, plans are in place to design a new focal-plane detector with increased position resolution (~ factor of 4) and higher count rate capabilities (~ factor of 10) based on the multi-layer thick gaseous electron multiplier (M-THGEM) technology and a highly segmented anode pad matched to the M-THGEM design. The M-THGEM-based design also allows us to detect heavier ions opening new possibilities for experimental studies with heavy-ion beams from the combined Tandem+LINAC accelerator system at the FSU John D. Fox Laboratory.
The microscopic structure of the Pygmy Dipole Resonance
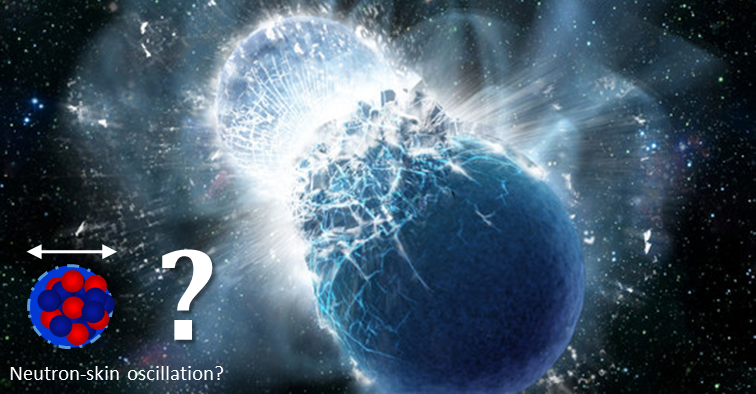
Neutron-star mergers (picture credit: NASA/Swift/Dana Berry) are discussed as one of the main sites for the rapid neutron-capture process. Different nuclear physics inputs shape the final isotopic abundance pattern. A low-lying dipole mode of the atomic nucleus, possibly corresponding to a dipole-type oscillation of the neutron skin (compare lower left corner), strongly influences neutron-capture rates.
Theoretical studies have shown that the excitation strengths to 1- states below and around the neutron-separation threshold critically influence neutron-capture rates in explosive stellar scenarios. Not including the low-lying electric dipole strength, also often denoted by Pygmy Dipole Resonance (PDR), can lead to variations of the capture rates by up to a factor of thousand. It is, however, not clear whether the strength, i.e. γ-ray strength function, will be the same in the excitation and decay channels. More experimental data are needed to study the γ-ray strength function in both channels and to understand the microscopic origin of the PDR.
A unique structure change was predicted in stable fp-shell nuclei, which is expected to influence the PDR strength. A sudden increase of the excitation strength has already been observed. If a connection to an underlying structure change can be experimentally proven, important and new information for the formation of this strength in neutron-rich nuclei far off stability would be obtained.
Using the Super-Enge Split-Pole Spectrograph in combination with the CeBrA γ-ray detectors, (d,p) singles and (d,pγ) coincidence experiments will be performed to study the single-particle character and γ-decay behavior of excited 1- states in the fp shell.
Alpha clustering and its connection to enhanced E1 strength - Possible implications for (γ,α) reactions on rare-earth nuclei
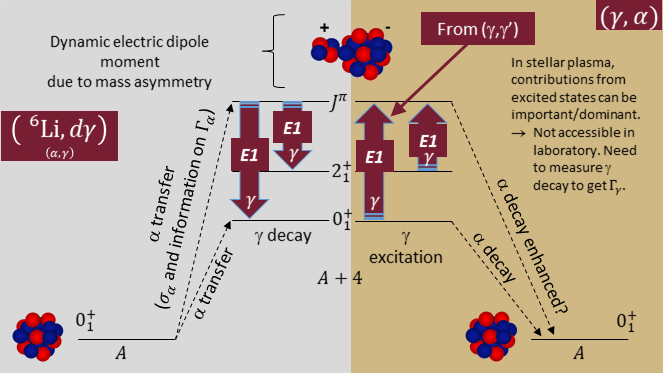
α-cluster states can be populated with the (6Li,d) α-transfer reaction. Their γ decay can be studied in coincidence with the residual deuterons. In combination with data from (γ,γ') experiments, information about the (γ,α) reaction, relevant for the p process, can be obtained.
As part of the p process, the rare-earth nuclei 144,146Sm and their neighbors are produced in photodisintegration reactions on seed nuclei, which were synthesized during the slow neutron-capture process (s process). (γ,α) reactions become increasingly important when approaching the N = 82 magic neutron shell closure because of the comparably low Qα values. However, direct measurements of this reaction on heavy nuclei are extremely challenging.
The goal of this project is to populate possible A+α structures in rare-earth nuclei via the (6Li,d) α-transfer reaction and to study their γ decay in coincidence with the residual deuterons, which will be detected with the Super-Enge Split-Pole Spectrograph. In a macroscopic picture, α-cluster states are expected to feature enhanced electric dipole (E1) strength due to their intrinsic mass asymmetry. If these states exist, their enhanced E1 strength will lead to a strong excitation with real photons and their pre-clustered structure to a larger α-decay width. Consequently, α-cluster states can strongly influence the outcome of (γ,α) reactions on rare-earth nuclei.
Research Projects for Undergraduate Students
Undergraduate students are welcome to apply for research internships. It is recommended that they have already passed Intermediate Modern Physics. Research projects, which are listed below, are currently available. Please contact Dr. Spieker directly if you are interested in one of the topics or if you want to get more information.
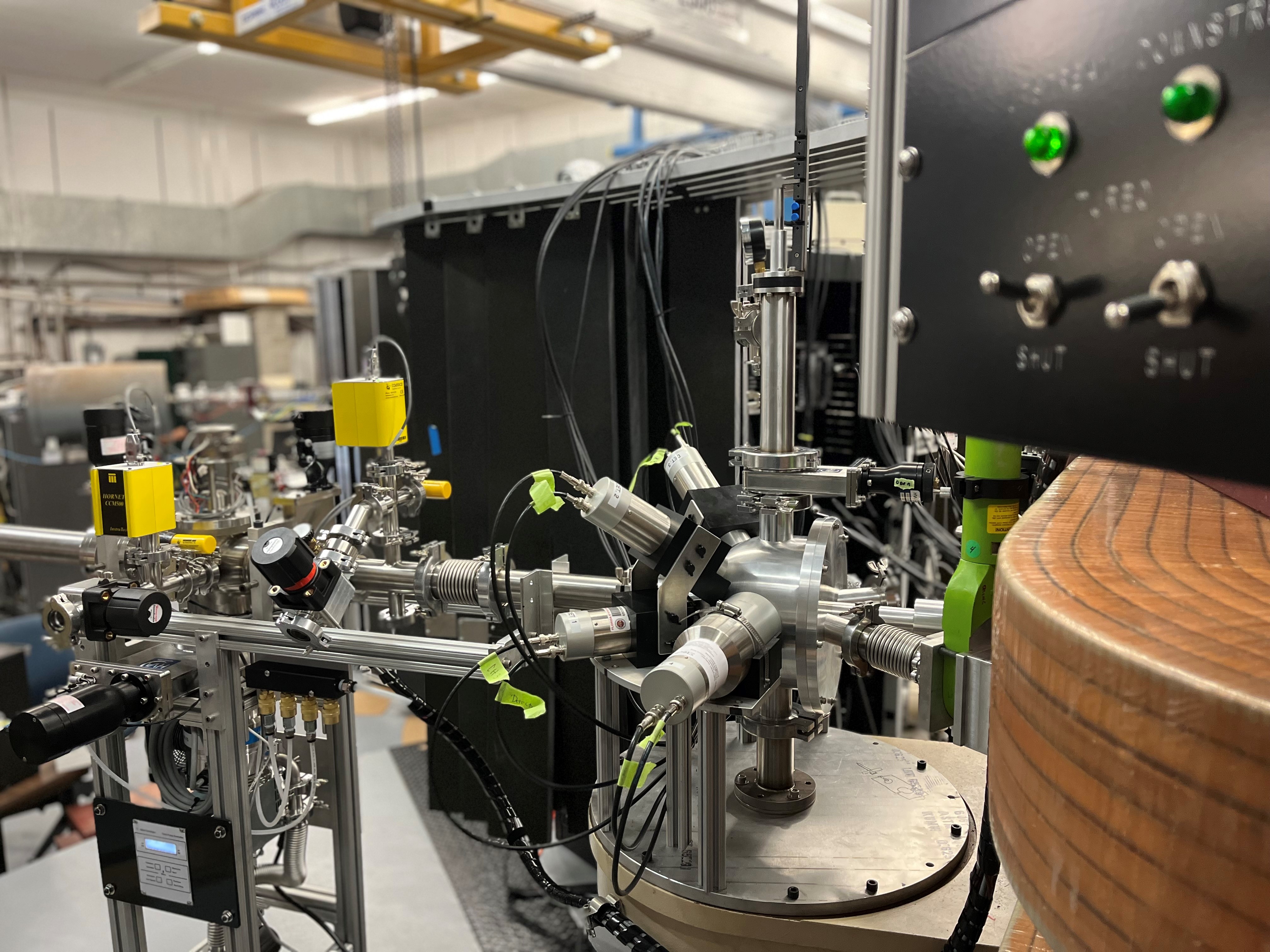
CeBr3 detectors around the new Gamma-target chamber at the FSU Super-Enge Split-Pole Spectrograph (CeBrA@SE-SPS).
Characterization of CeBr3 for γ-ray detection at the Fox Laboratory
γ-ray spectroscopy is an essential tool in Nuclear Physics and Nuclear Astrophysics. Our group has developed a new array, called CeBrA, for particle-γ coincidence experiments at the Super-Enge Split-Pole Spectrograph. This project will show you how γ rays interact with matter, how they can be detected, how to record data and how to analyze data coming from these detectors.
Once you have mastered these items, you will characterize the detectors thoroughly using different standard calibration sources and also learn how coincident detection of γ rays and particles works.
Data-analysis projects
Data-analysis projects are also available. The data were collected at the FSU Super-Enge Split-Pole Spectrograph as well as at the National Superconducting Cyclotron Laboratory of Michigan State University. If you want to know more about these possible projects, contact Dr. Spieker. Some programming skills are needed.

Short CV
Research fields: Nuclear structure, nuclear reactions, nuclear astrophysics, particle and gamma-ray spectroscopy.
In 2017, Dr. Spieker received the title of Dr. rer. nat. in Experimental Physics (PhD equivalent) from the Faculty of Mathematics and Natural Sciences of the University of Cologne in Germany. As part of the group of Professor Andreas Zilges, he performed particle-gamma coincidence experiments to study different aspects of nuclear structure. From November 2017 to October 2019, he worked at the National Superconducting Cyclotron Laboratory at Michigan State University as NSCL fellow with Professor Alexandra Gade. Dr. Spieker joined Florida State University as Assistant Professor in October 2019. In May 2020, Dr. Spieker received the FRIB (Facility for Rare Isotope Beams at Michigan State University) Visiting Scholar Award for Experimental Science. From 2020 to 2022, he served the GRETINA-GRETA User Executive Committee first as secretary and then as its chair. In 2022, Dr. Spieker was reelected for a second term (2022-2024) and also selected as a new member of the high-resolution in-beam gamma-ray spectroscopy group of the U.S. low-energy community.
email: mspieker at fsu.edu phone: (850)644-2066 office: Department of Physics, Keen Bldg., Room 217